Genetics is the study of genes: segments of information encoded in our DNA that are permanent and inheritable. To date, no living organism has survived without some form of genetic information, but how has genetic complexity evolved and how did this all begin?
The Role of DNA in the Cell
DNA (deoxyribonucleic acid) is a long molecule composed of ‘nucleotides’ which are small chemical structures as seen in Figure 1. There are four nucleotides, denoted by the letters A for Adenine, T for Thymine, G for Guanine, and C for Cytosine. Long chains of DNA interact with one another through chemical bonding (A:T and C:G are bonded pairs) and form the famous double helix structure.
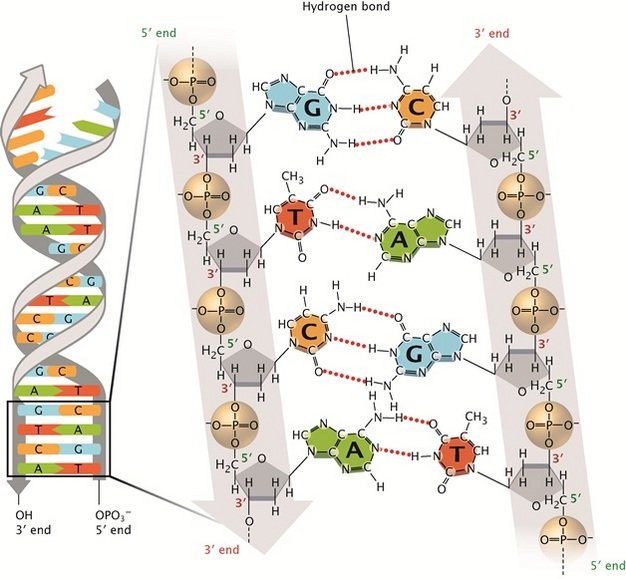
In order to actually express a gene, DNA must first be unwound from its helix and separated into two long strands. The individual nucleotides of one strand are then “read” by a protein complex called RNA Polymerase (polymer = a long-stranded molecule with repeating units, -ase = makes). This polymerase creates a new parallel strand called RNA, or ribonucleic acid (Figure 2).
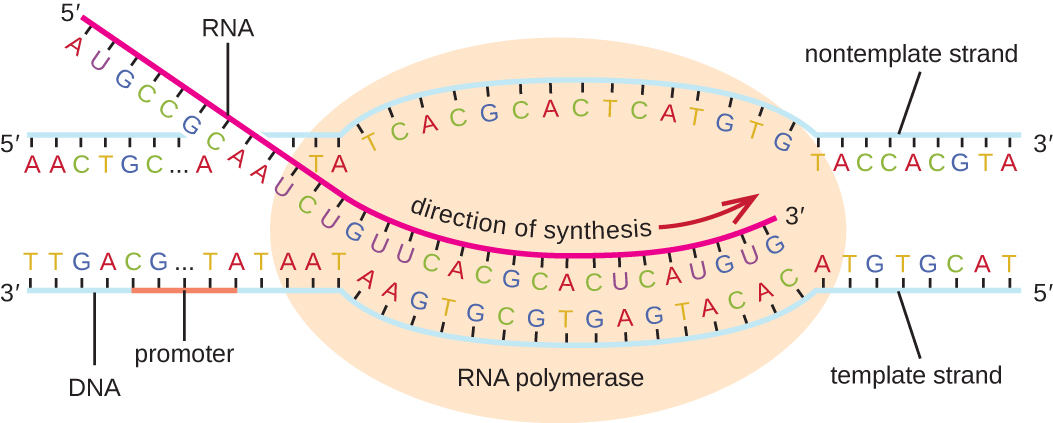
This name, and the RNA molecule itself, are highly similar to DNA. The name comes from the additional oxygen in the sugar structure in RNA, with DNA’s “deoxy” meaning “less oxygen” (Figure 2). The new RNA strand is a copy of the original DNA strand and follows the same nucleotide patterns. However, the RNA nucleotides are the opposite bonding partner of the original DNA nucleotides and therefore the RNA is nearly an exact copy of the original DNA’s partner strand in the helix (called the coding strand). If the original DNA strand contained a C, both the RNA and the coding strand would have a G in the same position. The main difference between the RNA strand from the DNA coding strand is that instead of a Thymine nucleotide, the RNA contains a different nucleotide called Uracil (U), pictured in Figure 3.
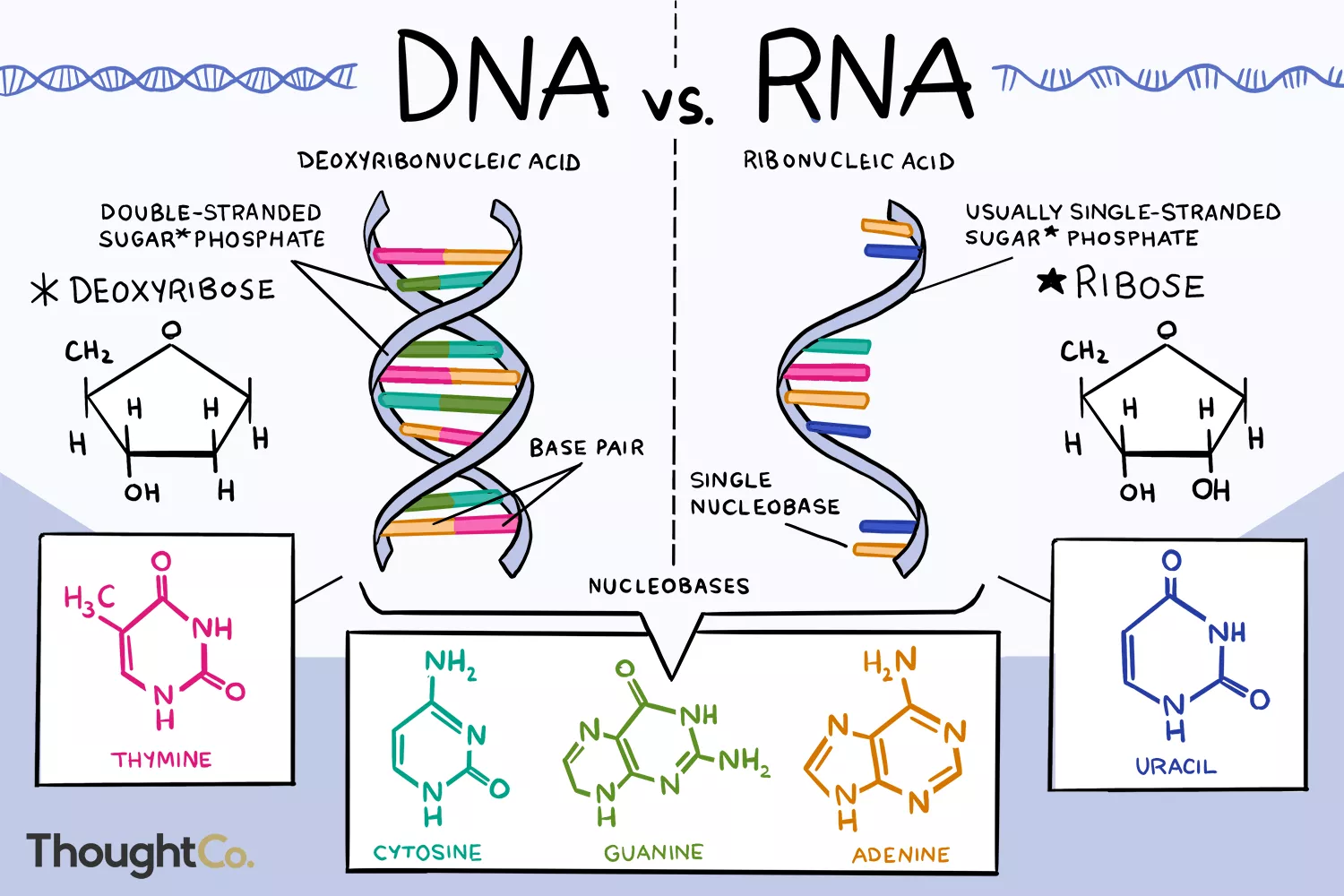
Once the RNA has been transcribed, it can leave the nucleus of the cell and enter the cytoplasm. There, a complex structure made up of RNA and proteins called the ribosome can translate the RNA molecule into a new protein molecule. Proteins go on to perform a wide variety of functions in the cell, including continuing to transcribe DNA and translate RNA.
The Chicken or the Egg: How was DNA first Transcribed?
DNA transcription is performed by proteins, which are themselves formed through RNA translation. A natural question arises: if DNA is transcribed by proteins and proteins come from DNA, how was DNA transcribed for the first time? This question gave many researchers pause. In the early days of DNA research before transcription was understood, Watson and Crick (who were initially credited with discovering the double helix structure of DNA) suggested that DNA might have the ability to transcribe itself. Within the next decade, the RNA Polymerase was discovered which proved that transcription is mediated by proteins and opened the initial transcription paradox. Yet, the 1982 discovery of RNA molecules that we now call ribozymes raised a possible answer. Ribozymes are RNAs with enzymatic activity – meaning they are capable of catalyzing chemical reactions, which potentially includes transcription and translation.
Since the 1960s, some scientists have been developing a theory about the origin of life called the “RNA world hypothesis”; perhaps the first living organisms stored genetic information in RNA, not DNA. DNA would require transcription to form RNA and then translation to form proteins. However, if RNAs already existed and had enzymatic activity, they may have been capable of producing proteins on their own. How and why might DNA have come into existence in this universe? DNA offered a type of stability that RNA inherently did not have. The additional oxygen present in the sugar of RNA makes it less stable than DNA and more likely to cleave its own strand into two. As a result, DNA can persist in the cell for a longer period of time. The Cytosine nucleotide can additionally be easily mutated into a Uracil nucleotide through spontaneous deamination reactions (Figure 4). This mutation would be undetectable in RNA due to the natural incorporation of Uracil as a nucleotide but would stand out in DNA where Thymine replaces Uracil. This critical difference means that this type of mutation is noticeable and correctable by the cell’s mutation repair mechanisms.

Although DNA has become the main source of genetic information in the modern age, it is important to recognize that the history of life on Earth has had a rich and complex origin. It has been suggested that before the RNA world, there may have been a precursor molecule that stored the genetic information for the earliest living things. If this is true, there may be an even simpler answer to what was genetically necessary for life at its creation. Nonetheless, evolution has demonstrated that survival is best served when genetic information is stable, and DNA has been the most stable option thus far. It is possible that in the far-off future DNA might be replaced by another type of molecule in an evolutionary leap, but it is difficult to imagine when or what that replacement would be.
Peer Editor: Bhavyaa Tyagi