The Goal
Terepthalic acid is a symmetric aromatic acid found in petroleum. Tens of millions of tons of terephthalic acid are used annually in one of the most common plastics, polyethylene terephthalate (PET), which is the plastic used for soda bottles and often used in polyester fabrics. Much work by Kyoung-Shin Choi at the University of Wisconsin–Madison has focused on the production of 2,5-furandicarboxylic acid (FDCA), a plastic building block that could replace terephthalic acid. What is great about this plastic building block is that it can be readily derived from manipulating the sugars that make up the cellulose in plants in a few steps. Cellulose is the most abundant plant polymer that can be found in nature, and its manipulation could provide access to a host of useful chemicals.
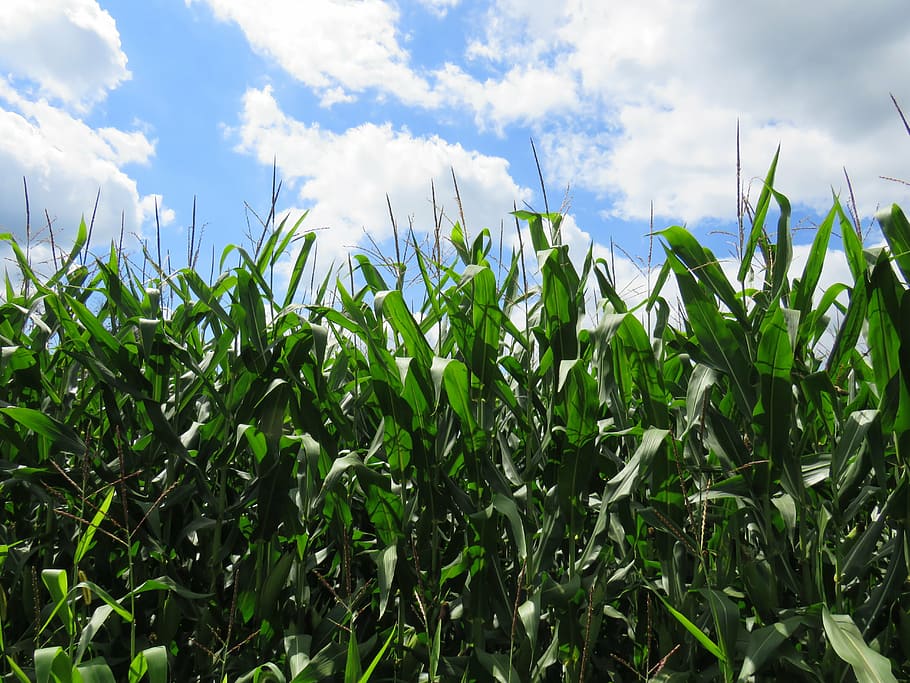
The Chemistry
The chemical steps involved in the transformation of cellulose to this plastic starting material involve abundant chemicals for catalysis and cheap electrode materials. This could offer a market alternative to petroleum-based plastic starting materials that could be produced by anyone in bulk. There are just two steps needed to convert cellulose to 2,5-furandicarboxylic acid. The first can be carried out in several ways, but a recent study used the relatively benign sodium bisulfate (NaHSO4) and zinc sulfate (ZnSO4) to convert cellulose to hydroxymethylfurfural (HMF) with a 53% yield after just an hour at 160 °C. What’s more, this method used two phases (meaning an oily phase that rested on top of water like salad dressing). The two phases allowed the catalytic components (chemicals that offer faster chemical pathways to products without being consumed), NaHSO4 and ZnSO4, to be separate from products and reused in subsequent reactions with only mild loss of activity.
Electrochemistry takes the HMF produced directly from cellulose and can convert it to the starting material for plastic FDCA. Depending on how acidic the solution is, the electrochemical conversion between HMF to FDCA can have current efficiencies between 50 and almost 100%, where current efficiency relates to how much charge transferred at the electrode was a result of FDCA production. The lower efficiencies are obtained at acidic pHs while the higher efficiencies are at very basic conditions (around pH 10 like a dilute solution of lye). The use of acidic conditions represents an achievement as the first reaction would be very acidic and being able to operate the electrochemistry from the aqueous phase of the first reaction would be quite industrially convenient. Additionally, FDCA becomes a solid at these acidic conditions, making isolation of the final product as easy as pouring off the liquid. For these reasons, while the lower yield may be disappointing, it may prove industrially cheaper to take that hit in yield and avoid the accumulation of wasted chemicals to adjust the pH drastically.

The Future
Bioplastics appear as an appealing alternative to petroleum-based plastics for several reasons to include petroleum independence, sustainability, and increased biodegradability. Importantly, these plastics must retain durability, resistance to fouling, and high melting points to be deployed immediately. It just so happens that a recent paper showed excellent accommodation of all these described requirements using a copolymer of FDCA and 1,20-eicosanediol. The other component also can be obtained from plants. This copolymer was hydrophobic and melted at 107 °C and despite these qualities could be degraded by unspecialized enzymes (or proteins that could be found in most organisms) with over 10% loss in mass over 21 days at 37 °C, meaning that it’s unlikely to persist in the environment as long as many bioplastics currently available such as polylactic acid.
In the face of these advances, bioplastics could feasibly escape limitations that exist with petroleum-based plastics. The two primary benefits seen here are universal access to plastic starting materials and biodegradability of these plastics. With these two qualities, applications that demand single-use plastic could be fulfilled while limiting their environmental impact. This is one of a number of examples of how scientists strive to establish a brighter future for the world through creative problem solving – brighter futures where grass clippings could become fine shirts and corn husks could bottle cola.
Peer Edited by Rachel Cherney