Much of the diverse matter in our naturally occurring world is made up of only a few chemical elements: carbon, hydrogen, oxygen, nitrogen, sulfur, and phosphorus. One of these elements, carbon, is the subject of study for an entire field of chemistry—organic chemistry. This might seem like an awful lot of energy directed at one element, especially when there are 117 other elements in the world. What makes carbon so special?
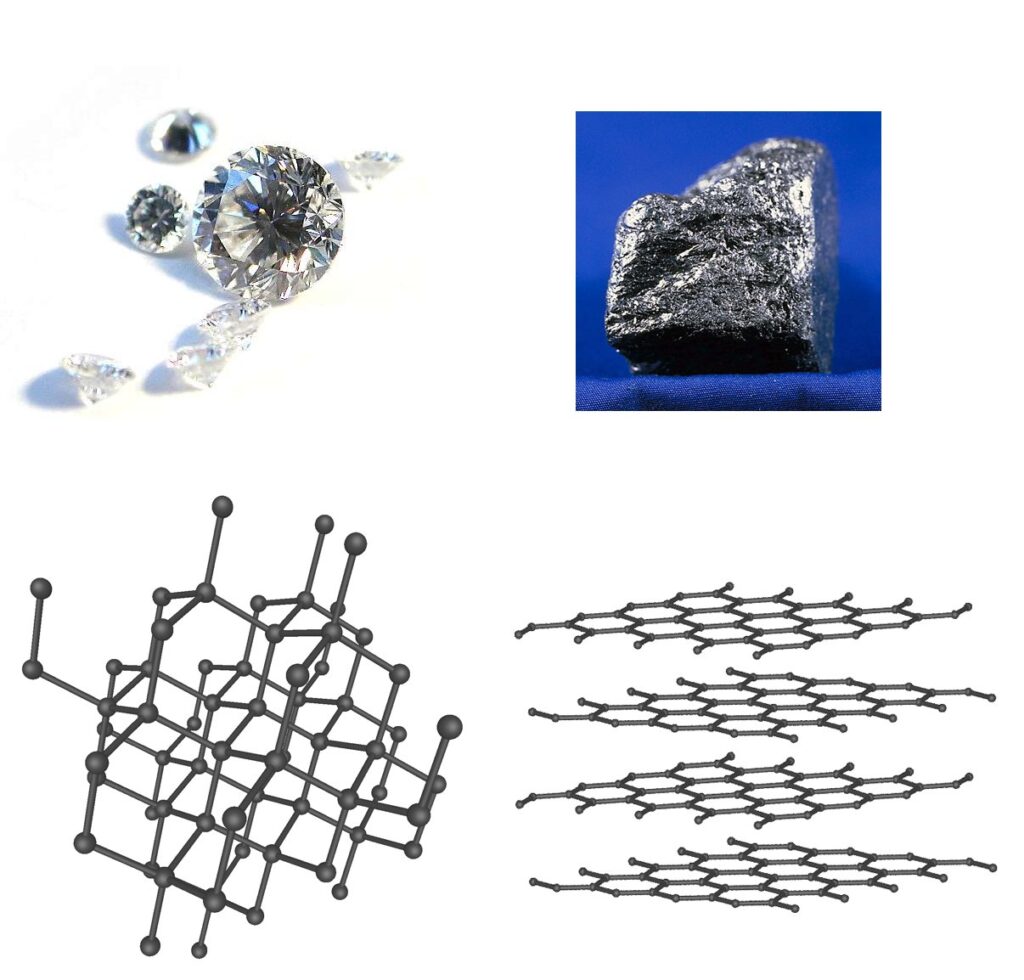
Put simply, life on earth would not be possible without carbon. Carbon is a unique element; a single carbon atom can form stable chemical bonds with four other atoms at a time. In contrast, oxygen can only stably form two bonds, and nitrogen three.
Carbon’s ability to form a high number of stable bonds with other elements and itself allows for a great deal of structural diversity that gives rise to all the complex molecules that make up living organisms. For instance, cell walls are made up of hydrocarbon (hydrogen and carbon) chains. Sugars, which give energy to many plants and animals, are made up of carbon and oxygen rings. Carbon is abundant in DNA, the medium that stores instructions for all life.
Living organisms also create many carbon-based compounds that are useful in medicine, materials technology, fragrance and beauty, and more. Because of this, chemical reactions that bond one carbon atom to another—carbon-carbon couplings—are particularly useful in synthetic organic chemistry.
Joining two carbon atoms together, however, can be a bit tricky to pull off. Any particular carbon atom in a shelf-stable compound is almost guaranteed to already have four bonds. With four bonds, a carbon atom is remarkably stable and relatively unreactive. To join two carbon atoms together, chemists must force a carbon to give up one of its bonds, making it highly reactive and susceptible to unwanted side reactions with water or air. As a result, chemists must be vigilant when setting up carbon-carbon couplings to ensure they contain only the specific chemicals needed.
Luckily, chemists have identified a useful tool in the field of carbon-carbon coupling research: metal catalysts. Metal catalysts help to create reactive carbon atoms and bring them close together, so they have a higher chance of coupling with each other.
The specific carbon-carbon coupling I performed, called a Negishi coupling, uses palladium as its metal catalyst. But, to explain how this reaction works, let’s think of it in terms of something many are familiar with: love and relationships.
Carbon-Carbon couples: a messy modern romance
The stage is set at the hottest new bar in town, the Round Bottom Flask. Carbon A enters bonded to four of its friends. Carbon A is a level-headed perfectionist, 3 months post-break up from an element it thought it’d be bonded to forever. Carbon A’s friend, Iodide A, convinced it to tag along on a night out. Iodide A was always a fun time, and certainly helped distract Carbon A from the pain of a breakup, but it wasn’t always the most dependable element around. Admittedly though, Carbon A was having a decent time so far bonding with its friends over drinks.

Zinc Inserts Itself
Just then, Zinc makes its entrance. Zinc is broody, has side bangs, and wears a leather jacket—a total element magnet. Immediately, Zinc notices Iodide A’s charismatic personality and starts chatting them up.
Iodide A can’t resist. Zinc is warm and bold, like a good cup of coffee. Zinc first inserts itself into Carbon A and Iodide A’s conversation, then between their bond. Before long, Iodide A is mesmerized by Zinc and completely ignores Carbon A. Carbon A tries to regain Iodide A’s attention, but to no avail. Iodide A and Zinc become a pair, leaving Carbon A upset, unstable, and reactive.
Palladium Catalyzes the Coupling
Seeking solace, Carbon A beelines to the bar and starts venting its woes to a total stranger, Palladium. Palladium pities Carbon A’s desperation and decides to help. It scans the room looking for potential elemental matches and spots our second carbon, Carbon B.
From across the room, Carbon B looks perfectly content, happily bonded to four other atoms. However, Palladium notices one of these bonds is to another iodide, Iodide B. As we know, iodides can be flaky—presenting the perfect opportunity.
Palladium and Carbon A make their way over to Carbon B’s compound. Palladium briefly disrupts Iodide B’s bond to whisper that Zinc—tall, dark, and handsome—just arrived at the Round Bottom Flask. Iodide B looks over at Zinc and swoons.
A Carbon-Carbon Bond Forms
With Iodide B distracted, Palladium steps in as a first-class wing-element and introduces Carbon A to Carbon B. Turns out, Carbon B is single, emotionally available, and looking for love. Carbon B complements Carbon A’s natural abundance and a connection immediately forms between the two. They become engrossed in conversation, forming a strong, stable bond.
With the carbons newly bonded, Palladium smiles—patting itself on the back for a job well-done—and quietly returns to the bar. There, it sips a drink next to an empty seat, patiently waiting for another distressed carbon atom to come along.
(There were, of course, some liberties taken with the actual chemical mechanism to tell this story. I hope chemists and soap opera screen writers alike can forgive me, though.)
—
Bree Iskandar is a pharmaceutical sciences Ph.D. candidate at the University of North Carolina at Chapel Hill and is passionate about making and communicating scientific discoveries. Find her at breezyscience.com or follow her on Twitter @breezyscience.
Peer Editor: Corban Murphey